by Roberto de Jesús Avena-Bustillos and Eli Terán-cabanillas
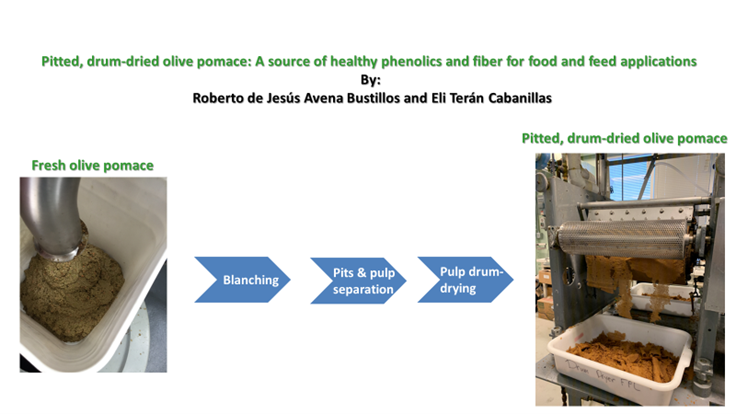
Olive oil is relevant, especially for its excellent nutritional properties; however, a large amount of waste and byproducts is produced during its production and is considered a serious environmental problem[i]. Olive pomace (OP) is the main by-product (80% of olive fruit) in olive oil production, obtained by expelling olive oil from the pulp in one or multiple extraction steps without any chemical treatment [ii]. OP is a heterogeneous biomass composed of a significant amount of moisture, fiber and oil, and its chemical composition may vary depending on regional crop conditions, fruit quality, technological processes, including multiple oil extraction steps, and storage[iii].
OP is mainly composed of water (70%–75%), polysaccharides (lignin, cellulose, hemicelluloses, pectins, and oligosaccharides), pigments, fatty acids, proteins, and phenolic compounds [iv],[v]. The main functional properties of OP are related to its high polyphenol content, also known as natural antioxidants, and high concentrations of dietary fiber. Hydroxytyrosol is considered the main phenolic compound of OP[vi] and is considered a health-promoting phenolic compound, as several studies have indicated it has antioxidant, anti-proliferative, and anti-inflammatory activities, and beneficial effects by preventing oxidative stress on the cardiovascular system and promising effects against diabetes, inflammation, nervous disorders, angiogenesis, cancer, heavy metal toxicity, hemolysis, LDL oxidation, muscle damage, and nephrotoxicity[vii],[viii].
The development of foods rich in bioactive compounds such as fiber and phenolic compounds has become a novel trend in the food industry. The addition of common foods with olive by-product compounds can improve the nutritional profile of products or the stability of food matrices and contribute to consumers health benefits. We evaluated mice health benefits of drum-dried pitted OP pulp obtained after first and second olive oil extraction. For that, fresh OP was steam blanched, then pits and skins separated in a pulper/finisher, and pulp drum-dried and milled. OP was characterized by proximate analysis, total soluble phenolics (TSP), individual phenolics, and dietary fiber. Drum-dried pitted OP from first and second extraction was formulated at 10% and 20% in a high-fat mice diet. Low fat (5%) and high fat (18%) control diets were used for comparison
OP from the first oil extraction had a higher fat, protein, and ash (minerals) than OP from the second extraction. Drum-dried pitted OP from second oil extraction had a higher total and insoluble fiber content (Table 1).
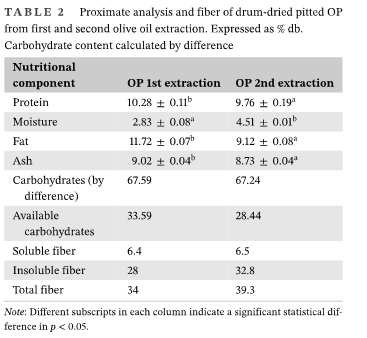
The three main phenolics in the drum-dried pitted OP from first oil extraction were hydroxytyrosol, vanillin, and verbascoside, while from second oil extraction were vanillin, hydroxytyrosol, and vanillic acid, in that order from higher to lower concentrations. Hydroxytyrosol was more than 50% depleted in dried OP from first to second oil extractions. Higher water expelling during the first oil extraction and longer enzymatic action to the time for the second oil extraction step can explain these differences in phenolic concentration and composition. The phenolic characterization combined with the TSP and AC measurements served for comparing the effect of phenolics on weight gain and intestinal microbiota balance in mice from various diet formulations, indicating that the use of drum-dry pitted OP in mice diets reduced mice liver fat significantly, likely due to the high phenolic content found in OP.
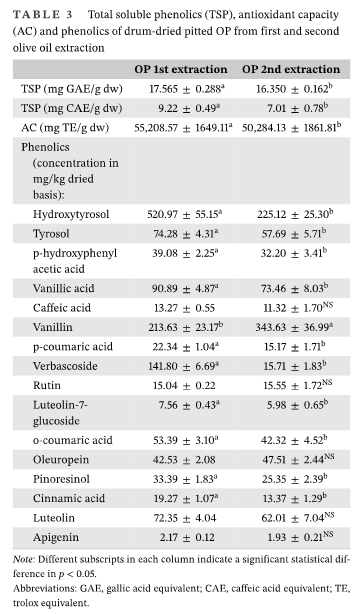
After five weeks of feeding mice with different diets, weight gain was higher in the high-fat control group, but there were no differences from the low-fat control group and the OP first and second extraction at 10%, while mice fed with the high-fat OP diets first and second extraction at 20% had a lower weight (Figure 1). A higher soluble fiber content on diets with 20% drum-dried pitted olive pomace (either from the pomace of first or second oil extractions) could explain the lower weight gain in mice consuming these two diets. After mice necropsy, the weight of adipose tissue was higher for the high-fat diet compared to the high-fat 10% and 20% OP diets and the low-fat control diet, but no significant differences for the low-fat control diet and the high-fat with 10% OP diets. Adipose tissue gain was the lowest with the 20% OP diets (Table 3) and this result is correlated to lower mice weight gain as reported in Figure 1.
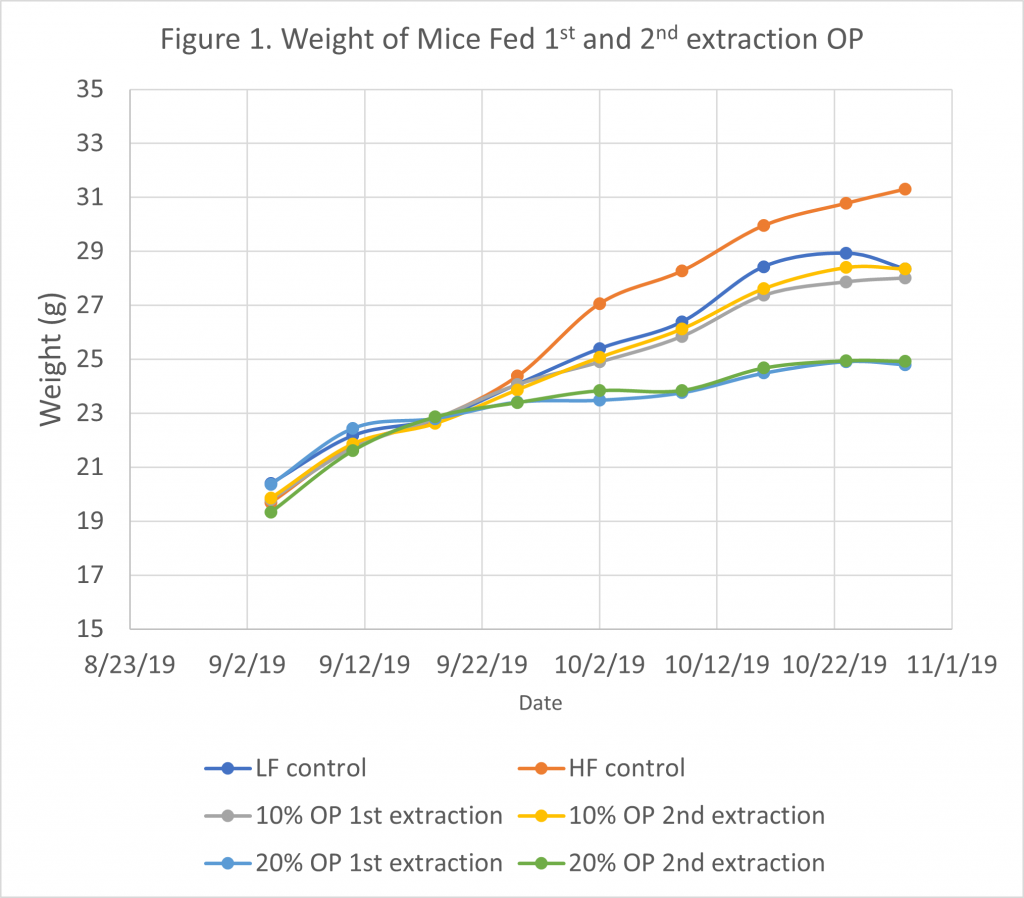
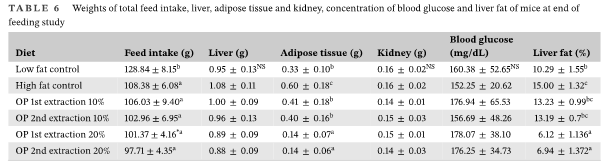
After feeding mice with OP diets, we analyzed gut microbiota and an increased abundance of Firmicutes was found, compared to the LF diet and HF diet (Figure 2). Composition of bacterial diversity appears to change between thin and obese, increasing the number of Bacteroidetes and decreasing Firmicutes in obesity and type 2 diabetes[i]. Bacteroidetes increased about four times with OP diets. Most research associates Bacteroidetes with adequate body weight and have a positive correlation with reduced body fat[ii]; this could be related to the decrease of this phylum in mice consuming a high-fat diet compared to those consuming OP diets. The Firmicutes/Bacteroidetes ratio has been related with obesity, where relative abundance of Firmicutes is usually increased and the Bacteroidetes, decreased. However, we observed that in our groups with OP diets there was an increment in both Firmicutes and more pronounced one for Bacteroidetes, resulting in lower Firmicutes/Bacteroidetes ratios as found previously in hamsters fed Chardonnay grape seed flour[iii].
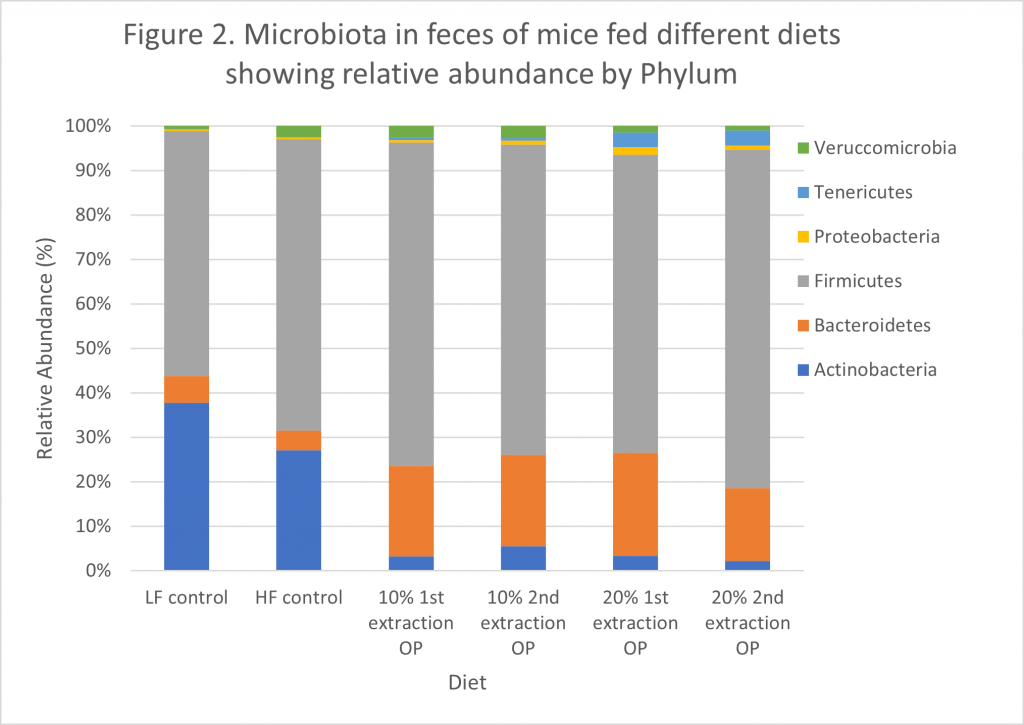
Conclusions: Drum-dried pitted OP obtained from first oil extraction operation has higher soluble phenolics and antioxidant capacity and less insoluble fibers when compared to using pomaces from second oil extraction operation. Higher soluble fiber and phenolic composition of drum-dried pitted OP promoted less weight gain and healthy microbiota balance in mice. These findings suggest that the beneficial effects of drum-dried pitted OP on harmful high-fat diets may induce healthy modification of intestinal microbiota and metabolic processes.
Roberto de Jesús Avena-Bustillos Eli Terán-cabanillas
[i] Castaner, O., Goday, A., Park, Y., Lee, S., Magkos, F., Shiow, S. T. E., & Schröder, H. (2018). The gut microbiome profile in obesity: A systematic review. International Journal of Endocrinology, 2018, 1– 9.
[ii] Crovesy, L., Masterson, D., & Lopes, E. (2020). Profile of the gut microbiota of adults with obesity: A systematic review. European Journal of Clinical Nutrition, 74(9), 1251–1262.
[iii] Kim, H., Kim, D. H., Seo, K. H., Chon, J. W., Nah, S. Y., Bartley, G. E., Arvik, T., Lipson, R., & Yokoyama, W. (2015). Modulation of the intestinal microbiota is associated with lower plasma choles- terol and weight gain in hamsters fed chardonnay grape seed flour. Journal of Agricultural and Food Chemistry, 63(5), 1460–1467.
[i] Nunes, M. A., Costa, A. S. G., Bessada, S., Santos, J., Puga, H., Alves, R. C., Freitas, V., & Oliveira, M. B. P. P. (2018). Olive pomace as a valuable source of bioactive compounds: A study regarding its lipid- and water-soluble components. Science of the Total Environ- ment, 644, 229–236
[ii] Cardoso, S. M., Silva, A. M. S., & Coimbra, A. (2002). Structural characterization of the olive pomace pectic polysaccharide arabinan side chains. Carbohydrate Research, 337, 917–924.
[iii] Bosco, A. D., Mourvaki, E., Cardinali, R., Servili, M., Sebastiani, B., Ruggeri, S., & Castellini, C. (2012). Effect of dietary supplementation with olive pomaces on the performance and meat quality of growing rabbits. Meat Science, 92(4), 783–788.
[iv] Lin, S., Chi, W., Hu, J., Pan, Q., Zheng, B., & Zeng, S. (2017). Sensory and nutritional properties of Chinese olive pomace based high fiber biscuit. Emirates Journal Food and Agriculture, 29(7), 495– 501
[v] Rodríguez, G., Duthie, G. G., Wood, S., Morrice, P., Nicol, F., Reid, M., & Fern, J. (2012). Alperujo extract, hydroxytyrosol, and 3, 4-dihydroxyphenylglycol are bioavailable and have antioxidant properties in vitamin E-deficient rats—A proteomics and network analysis approach. Molecular Nutrition Food Research, 56, 1131– 1147.
[vi] Sinrod, A. J. G., Avena-Bustillos, R. J., Olson, D. A., Crawford, L. M., Wang, S. C., & Mchugh, T. H. (2019). Phenolics and antioxidant capacity of pitted olive pomace affected by three drying technolo- gies. Journal of Food Science, 1–9.
[vii] Hu, T., He, X. W., Jiang, J. G., & Xu, X. L. (2014). Hydroxytyrosol and its potential therapeutic effects. Journal of Agricultural and Food Chemistry, 62(7), 1449–1455.
[viii] Wani, T. A., Masoodi, F. A., Gani, A., Baba, W. N., Rahmanian, N., Akhter, R. R., Wani, I. A., & Ahmad, M. (2018). Olive oil and its principal bioactive compound: Hydroxytyrosol–A review of the recent literature. Trends in Food Science & Technology, 77, 77–90.