By Matteo Pietraccini
The Mediterranean area is an undeniable bottomless source of treasures, that provides us with magnificent products. Among others, stands the olive tree. This ancient plant was likely present on Earth since long ago, as some fossilised leaves found in Mongardino (Italy) date back to Pliocene [1], and now it is exploited worldwide for its precious fruits to obtain olive oil. Olive oil is a fundamental brick in the Mediterranean diet and its production process represents an undeniable asset for several countries that look out in this region (as depicted in Figure 1).

It can be obtained with different processes: the traditional way, the two- and three-phase flow centrifugation ways. All three include a pressing step, while only the last two include a centrifugation step. Moreover, water is used in the three-phase process, which mechanically helps the separation of the oil particles from the solid residue. In average, 100 kg of olives lead to about 60 kg of olive oil, which intrinsically generates about 40 kg of wastes, constituted by foreign matter (leaves, branches, soil), water (to wash and/or in the centrifugation step) and olive pomace (the solid residue, made of olive pit, skin and pulp).
The will to apply the concept of circular economy to the already existing processes is more and more on everyone’s agenda. The olive oil production process is no exception, so several works were developed to exploit olive pomace (as in Eliche-Quesada et al (2016) [2], Yucel (2011) [3] and Akar et al (2009) [4]), making it a departure point instead of something to dispose of. However, a deep knowledge of this resource must be achieved.
In Pietraccini et al (2021) [5], a sample of pelletized Spanish olive pomace (from a two-phase flow extraction process) was analysed and a characterisation method is proposed. The work aimed to fully characterise the sample in term of chemical composition and physical properties, as to define its likely behaviour as a solid fuel.
The step zero consisted in grinding and sieving the sample. A hammer mill and four sieves (45, 75, 212 and 425 μm) were chosen to fulfil the task. Four samples were thus obtained: A (the finest), B, C and D (the coarser). They are shown in Figure 2.

The solid-state 1H-NMR showed no important difference in the chemical composition of the samples. Carbohydrates, aromatics, carboxylic and aliphatic species classes were chosen as investigation tools to identify cellulose, hemicellulose and lignin, respectively, through 1H-Nuclear Magnetic Resonance (1H-NMR).
A thermo-gravimetric analysis (TGA) was employed to calculate the Volatile Matter (VM), the Fixed Carbon (FC) and the Ash content of the samples. The VM-to-FC ratio was used to represent the thermal reactivity of the sample. The values are reported in Table 1, compared to those reported in Miranda et al (2008) [6].

The Scanning Electron Microscope (SEM) imaging identified and investigated the sample behaviour to crushing and grinding, as well as its effect on the different tissue components (olive skin, pulp, pit and kernel), as on the morphology of generated particles. Figure 2 reports some of the most representative images of the four samples. Some structures and the supposed corresponding tissue in the olive were identified.
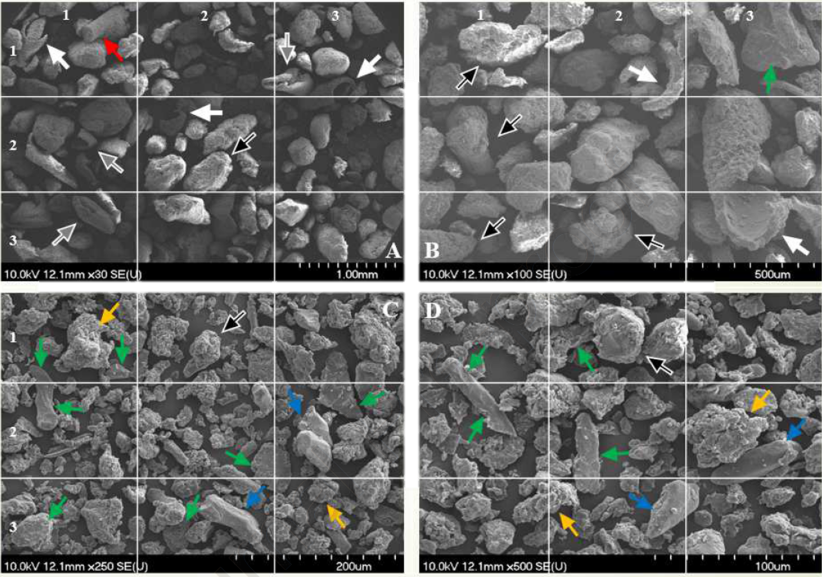

In conclusion, particle size did not have a major role in determining the thermal behaviour of the samples, but it seemed to be co-ruling over it with the heterogeneous nature of the sample itself. Thus, it seems that the grinding-sieving step had a significant influence on the sample obtained. The proximate analysis proved to be a reliable tool for estimating the thermal reactivity of olive pomace, especially the VM-to-FC ratio. In these terms, the fact that the sample came from a non-homogeneous matrix may lead to a deeply different thermal behaviour. For the purposes of a process that aims to thermally valorise a by-product, each step of the valorisation path must be considered, in order to prevent the process itself from being inefficient.
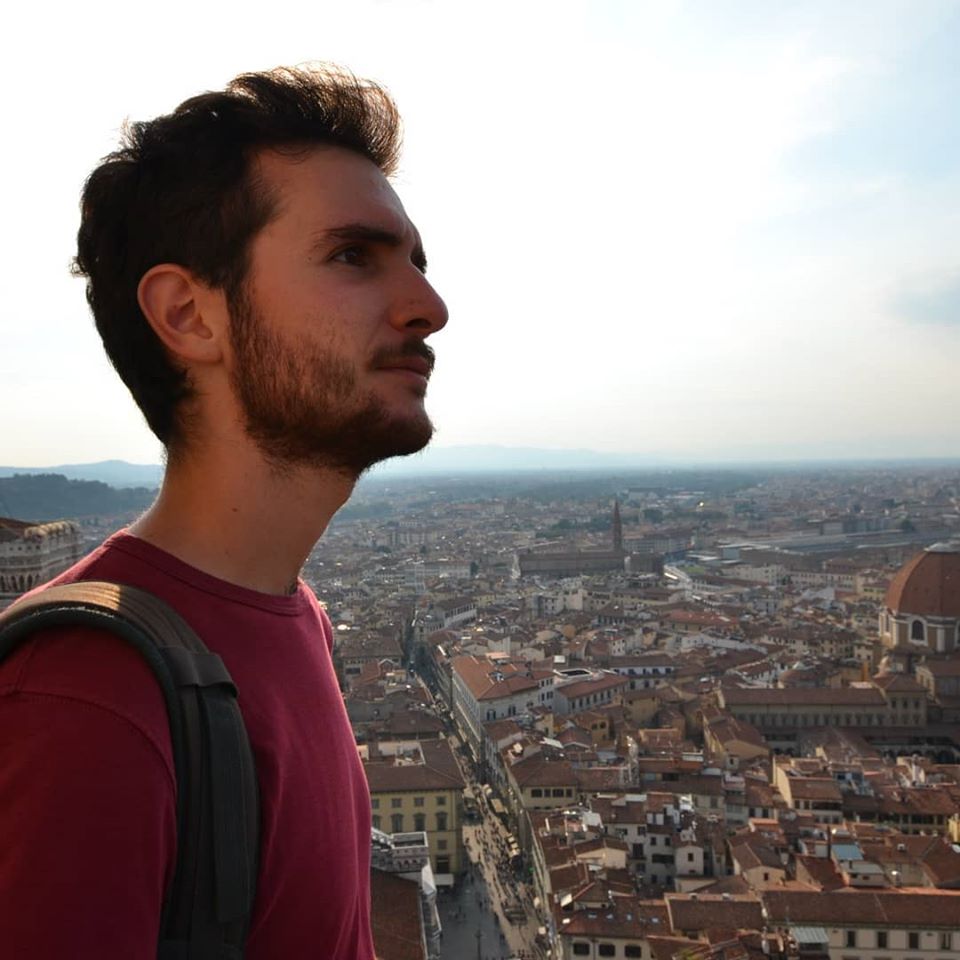
Matteo Pietraccini
Education
- Polytechnic of Turin – Chemical and food engineering (Turin, Italy)
- Polytechnic of Turin – Chemical engineering for sustainable processes (Turin, Italy)
- INSA Lyon – Energetic and Environment engineering (Lyon, France)
- Dalhousie University – Process engineering (Halifax, Canada)
Work experience
- R&D initiation project – INSA Lyon (Lyon, France). Development of an analytical characterisation method for olive pomace (BIOSOL project)
- Master thesis – Dalhousie University (Halifax, Canada). Study of the flammability and the explosion severity of an olive pomace sample
- R&D – Provademse (Lyon, France). Characterisation of the flow inside an anaerobic digester with long-distance interaction devices
- PhD – LRGP (Nancy, France). Determination of the phenomena involved in the fast thermal oxidation of organic powders
Papers
- Comparative analyses of three olive mill solid residues from different countries and processes for energy recovery by gasification – https://doi.org/10.1016/j.renene.2019.05.116
- Fast and tiny: A model for the flame propagation of nanopowders – https://doi.org/10.1016/j.jlp.2021.104503
- Effect of particle size distribution, drying and milling technique on explosibility behavior of olive pomace waste – https://doi.org/10.1016/j.jlp.2021.104423
References
- https://www.internationaloliveoil.org/olive-world/olive-tree/
- Eliche-Quesada D. and Leite-Costa, J. (2016). Use of bottom ash from olive pomace combustion in the production of eco-friendly fired clay bricks, Waste Management 48, 323-333.
- Yücel, Y. (2011). Biodiesel production from pomace oil by using lipase immobilized onto olive pomace, Bioresource Technology 102, 3977-3980.
- Akar, T., Tosun, l., Kaynak, Z., Ozkara, E., Yeni, O., Sahin E. N. and Tunali Akar S. (2009). An attractive agro-industrial by-product in environmental cleanup: Dye biosorption potential of untreated olive pomace, Journal of Hazardous Materials 166, 1217-1225
- Pietraccini, M., Danzi, E., Marmo, L., Addo, A., Amyotte, P. (2021). Effect of particle size distribution, drying and milling technique on explosibility behavior of olive pomace waste, Journal of Loss Prevention in the Process Industries 71, 104423
- Miranda, T., Esteban, A., Rojas, S., Montero I. and Ruiz, A. (2008). Combustion Analysis of Different Olive Residues, International Journal of Molecular Sciences, 9, 512-525
- Lanza B. and Di Serio M. G. (2015), SEM characterization of olive (Olea europaea L.) fruit epicuticular waxes and epicarp, Scientia Horticulturae 191, 49–56
- Lammi, S., Le Moigne, N., Djenane, D., Gontard N. and Angellier-Coussy H. (2018), Dryfractionation of olive pomace for the development of food packaging, Bio-composites, Industrial Crops & Products 120, 250-261
- Braadbaart, E. Marinova and A. Sarpaki (2016), Charred olive stones: experimental and archaeological evidence for recognizing olive processing residues used as fuel, Veget Hist Archaeobot 25, 415–430
- Marinova, E., van der Valk, M. A., Maria Valamoti S. and Bretschneider J. (2011), An experimental approach for tracing olive processing residues in the archaeobotanical record, with preliminary examples from Tell Tweini, Syria, Veget Hist Archaeobot 20, 471–478