By Frank H. Quina1
The anthocyanins are responsible for most of the red, blue and purple colors of fruits and flowers, in addition to some vegetables such as purple varieties of potatoes, corn, beans, onions and carrots, or red cabbage and some plant leaves such as purple trees, new foliage or the red leaves around poinsettia flowers or even autumn leaves (Figure 1). Although thousands of different anthocyanins have been characterized, almost all can be classified into six basic structural types that differ only in the number of hydroxy or methoxy substituents in the B ring (Scheme 1). In nature, the 3-hydroxy group of anthocyanins is always glycosylated, which is apparently important for thermal stability. Anthocyanins derived from malvidin-3-O-glucoside (Scheme 2) typically predominate in Vitis vinifera grapes, with lesser amounts of anthocyanins derived from cyanindin, peonidin, delphinidin and petunidin.
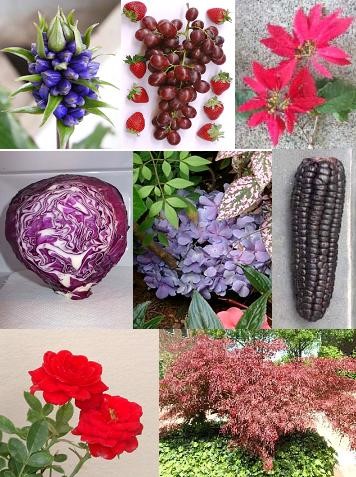
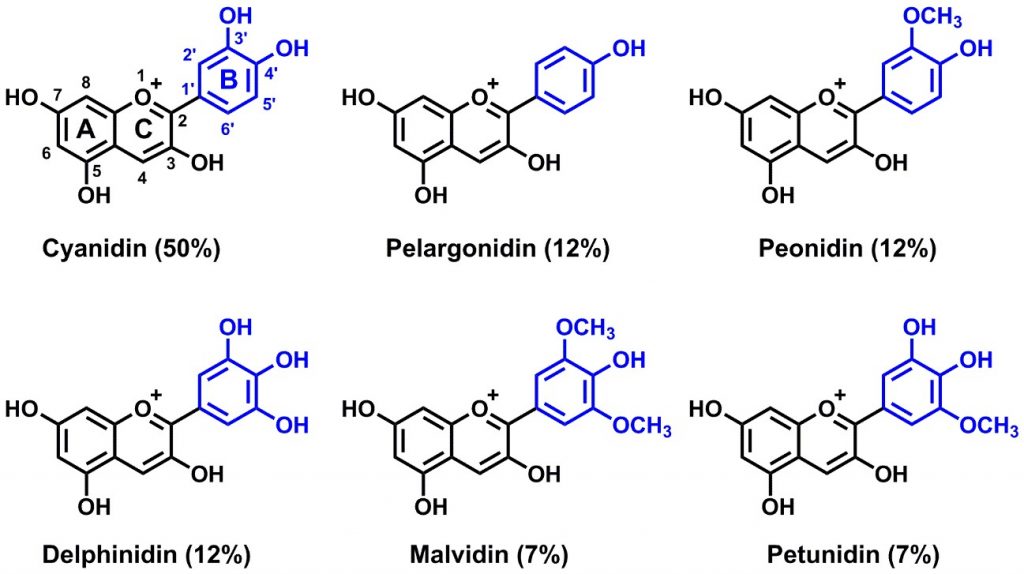
Anthocyanins accumulate in vacuoles of plants and have been proposed to play at least three important biological roles in plants: (1) as a color signal to attract pollinators to flowers or frugivores to fruit; (2) as an antioxidant; and (3) in the case of leaves, photoprotection of the photosynthetic apparatus against excess solar radiation. Although anthocyanins are safe to use as food colorants, with desirable antioxidant properties and other potential health benefits, their color is restricted to relatively acidic solutions (pH < 3). Especially around neutral pH, anthocyanins lose most of their color due to a pH-dependent reaction with water and a series of subsequent structural transformations.3 In this regard, red wines have managed to improve on Nature by slowly transforming the anthocyanins initially present in grape juice into a variety of more complex pigment molecules during the ageing of the wine. These complex reactions of the anthocyanins with colorless molecules and yeast metabolites present in the wine produce pyranoanthocyanins (Scheme 2), which are important contributors to the final color of the wine. Pyranoanthocyanins differ from anthocyanins by the presence of an additional pyran ring between the 4-carbon and the 5-hydroxy group of the anthocyanin precursor, which blocks the reaction with water, making the color of mature wines more pH-stable and much less susceptible to bleaching by additives such as sulfite than that of young wines. Pyranoanthocyanins also appear to contribute to the radical scavenging antioxidant capacity and to the organoleptic properties of aged red wines.
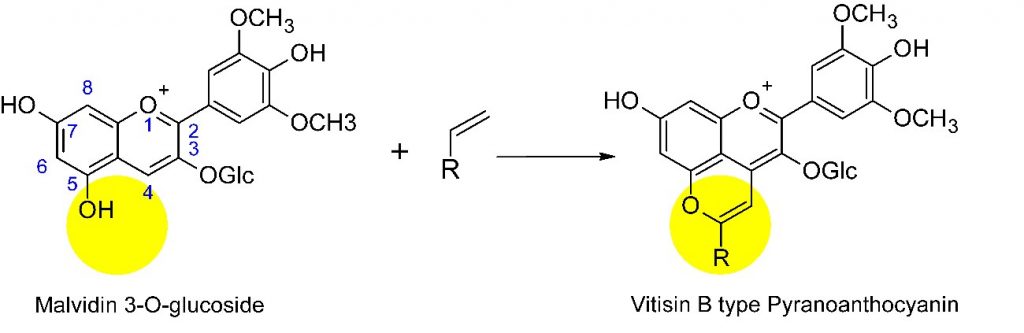
Over the last 15 or so years, we have collaborated with groups in Brazil, Portugal and the USA in studies of the photophysics of natural plant pigments, looking at what happens when these molecules absorb light.4 Although anthocyanins are commercially available, natural pyranoanthocyanins have to be isolated from the complex mixture of chemically distinct products present in mature wines. Consequently, we and others have concentrated on the study of pyranoflavylium cations (Scheme 3), molecules that are structurally analogous to natural pyranoanthocyanins but that can be expeditiously synthesized in the laboratory.5

Both anthocyanins and pyranoflavylium cations are weak acids, pyranoanthocyanins (pKas ca. 3.5-4.5) being slightly more acidic than anthocyanins (pKas ca. 4-5.5, or about as acidic as acetic acid). Upon absorption of light, phenols are known to become much more acidic, and this indeed happens with these plant pigments. When anthocyanins absorb light, the pKa of the resulting electronically excited state decreases to about -1 (almost as acidic as nitric acid). Pyranoflavylium cations also become more acidic when they absorb light, but the change in acidity is less than that of anthocyanins (pKas of excited pyranoflavylium cations are in the range of ca. 0.5). Within about 20 picoseconds (1 ps = 10-12 s = 0.3 mm at the speed of light), the initially formed electronically excited state of the anthocyanins in grapes transfers a proton to water to form the corresponding excited conjugate base; within another 200 ps, the excited conjugated base converts the absorbed light into heat and reprotonates, regenerating the original anthocyanin (Scheme 4). Pyranoflavylium cations take slightly longer (about a nanosecond or 10-9 s), but undergo a completely analogous cyclic excited state proton transfer process.6,7
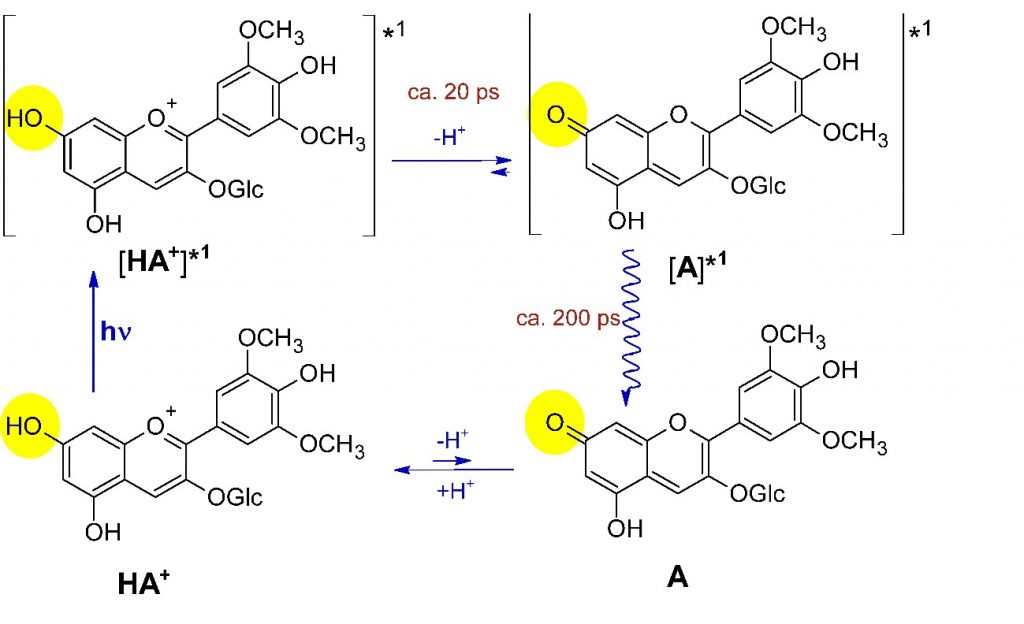
Anthocyanins also form complexes with colorless electron-rich “copigments” molecules such as hydroxybenzoic or hydroxycinnamic acids. Intramolecular copigmentation complexes are also present in acyl anthocyanins with one or more copigment molecules covalently attached to the sugar residues. The charge-transfer interactions involved in the copigmentation not only help stabilize the cationic form of the anthocyanin chemically, but also open up a new charge-transfer mediated channel for direct conversion of the absorbed light energy into heat that is even faster ( 1 ps) than excited state proton transfer. As far as we know, there are no published studies of copigmentation of pyranoanthocyanins, but it is reasonable to expect that it should occur.
Taken together, these two pathways, i.e., excited state proton transfer in uncomplexed anthocyanins or pyranoanthocyanins and ultra-rapid direct deactivation of the excited state in copigmented anthocyanins, contribute to make the color of anthocyanins and pyranoanthocyanins quite resistant to fading in sunlight. In current research in the laboratory, we are investigating additional types of light-induced chemistry that can occur in pyranoflavylium cations when the excited-state proton transfer pathway is blocked.8

Dr. Frank Herbert Quina (quina@usp.br) is a full professor at the Instituto de Química, Universidade de São Paulo, in São Paulo, Brazil. He received his Ph.D. from CALTECH (1973) and immigrated to Brazil in 1975 after a post-doctoral stint at the University of North Carolina, Chapel Hill. He is a Level 1A research fellow of the Brazilian National Research Council (CNPq), a fellow of the Royal Society of Chemistry, IUPAC and the Inter-American Photochemical Society, and a member of the Brazilian Academy of Sciences. He was a Senior Editor of Langmuir (2013-2016) and is currently an Associate Editor of ACS Omega and on the editorial boards of Photochemical and Photobiological Sciences, Photochemistry and Photobiology and the Brazilian Journal of Chemical Engineering. His group maintains active international research collaborations with groups in Portugal, Chile, Canada, China and the USA in the areas of the chemistry and photochemistry of natural plant pigments and the structure and dynamics of self-assembling colloidal systems. He is the fifth generation of his family with a registered cattle brand and the third generation to grow tropical fruit.
ORCID ID – https://orcid.org/0000-0003-2981-3390
References:
- For a more detailed discussion of current reseach on these plant pigments and leading references, see: Quina, F. H.; Bastos, E. L. Chemistry Inspired by the Colors of Fruits, Flowers and Wine. Anais da Academia Brasileira de Ciências, 2018, 90, 681-695. http://dx.doi.org/10.1590/0001-3765201820170492
- Kodama, M.; Tanabe, Y.; Nakayama, M. Analyses of coloration-related componentes in Hydranges sepals causing color variability according to soil conditions. The Horticulture Journal, 2016, 85, 372-379. http://dx.doi.org/10.2503/hortj.MI-131
- For a nice illustration of the pH-induced changes in the color of grape anthocyanins, see Figure 6 of: Terci, D. B. L.; Rossi, A. V. Indicadores Naturais de pH: Usar Papel ou Solução? Química Nova, 2002, 25, 684-688. http://quimicanova.sbq.org.br/detalhe_artigo.asp?id=5428
- Silva, V. O.; Freitas, A. A.; Maçanita, A. L.; Quina, F. H. Chemistry and photochemistry of natural plant pigments: the anthocyanins. Journal of Physical Organic Chemistry, 2016, 29, 594-599. https://doi.org/10.1002/poc.3534
- Silva, C. P.; Pioli, R. M.; Liu, L.; Zheng, S.; Zhang, M.; Silva, G. T. M.; Carneiro, V. M. T.; Quina, F. H. Improved Synthesis of Analogues of Red Wine Pyranoanthocyanin Pigments. ACS Omega, 2018, 3, 954-960. https://doi.org/10.1021/acsomega.7b01955
- Freitas, A. A.; Silva, C. P.; Silva, G. T. M.; Maçanita, A. L.; Quina, F. H. From Vine to Wine: Photophysics of a Pyranoflavylium Analog Of Red Wine Pyranoanthocyanins. Pure and Applied Chemistry, 2017, 89, 1761-1767. https://doi.org/10.1515/pac-2017-0411
- Freitas, A. A.; Silva, C. P.; Silva, G. T. M.; Maçanita, A. L.; Quina, F. H. Ground and Excited State Acidity of Analogs of Red Wine Pyranoanthocyanins. Photochemistry and Photobiology, 2018. https://doi.org/10.1111/php.12944
- Silva, G. T. M.; Thomas, S. S.; Silva, C. P.; Schlothauer, J. C.; Baptista, M. S.; Freitas, A. A.; Bohne, C.; Quina, F. H. Triplet Excited States and Singlet Oxygen Production by Analogs of Red Wine Pyranoanthocyanins. Photochemistry and Photobiology. 2018. https://doi.org/10.1111/php.12973